Toward a reduced CO2 footprint: The role of the chemical industry
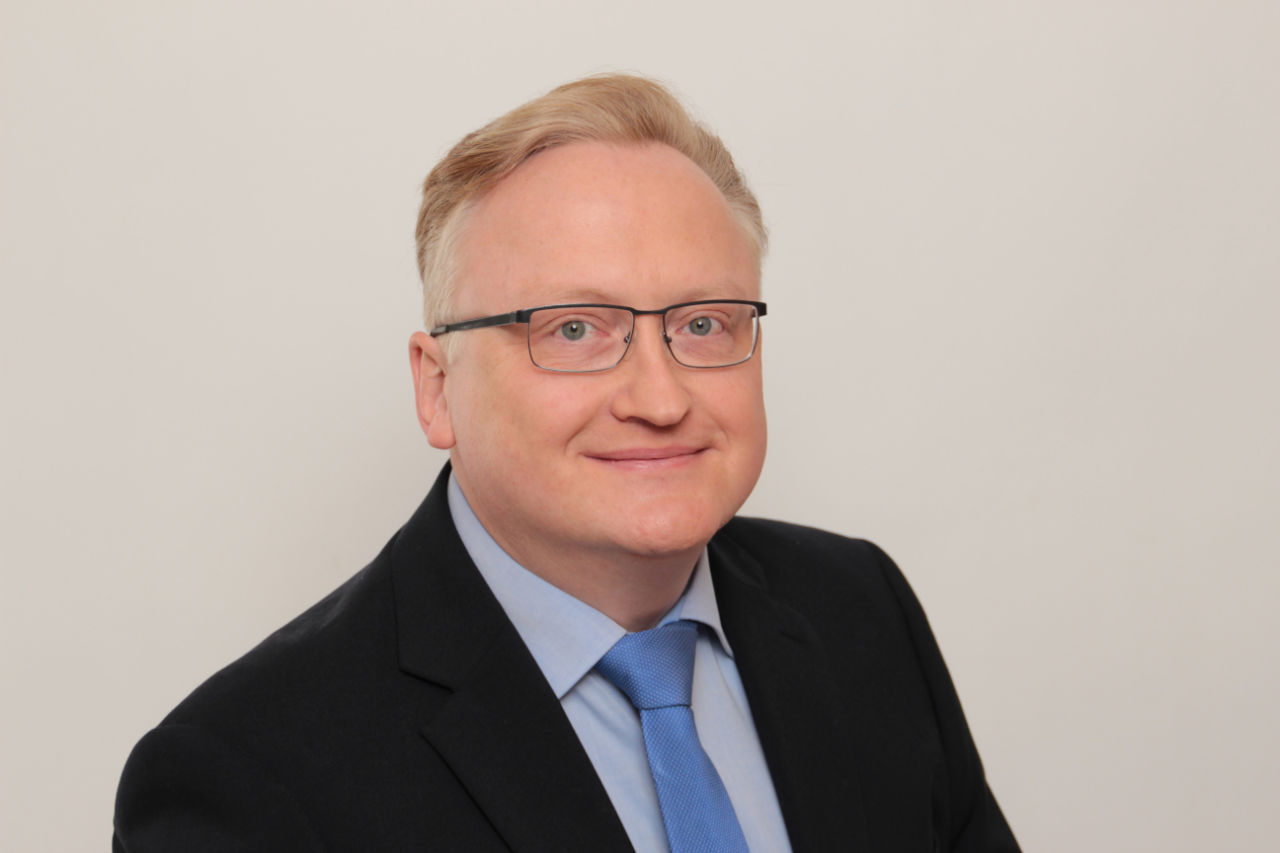
Interview with Peter Kolb, Senior Scientist at hte GmbH
As a chemist with hte for over 20 years now, what’s the most exciting aspect of your work?
During my training as a chemist, I became more and more fascinated by the fact that chemical processes and their products are an integral part of our daily life. Modern agriculture, pharmaceutical production, fuels, and construction materials would not be possible without a deep understanding of the chemical processes involved. Although chemical processes are sometimes regarded as “inherently dirty” by the public, the opposite is true: the chemical industry is a strong pillar of our well-being.
I joined hte nearly 20 years ago, and I was amazed about the potential to accelerate R&D in various crucial fields of chemical research. Now, we can offer our customers a broad range of highly optimized tools and workflows to help them explore novel processes and optimize the existing ones in order to give them a crucial competitive edge to cope with the challenges that lie ahead.
Currently, the chemical industry is undergoing significant changes. What is the biggest motivator and challenge?
In the course of the past several years, there has been an increasing public awareness toward climate change that is associated with the increasing levels of CO2 released in the atmosphere. Recently, the level of CO2 in the atmosphere has exceeded 400 ppm for the first time in history, and it is now generally agreed that high levels of CO2 make extreme weather conditions more likely. A few weeks ago, several parts of western Germany saw periods of extreme rainfall, leading to dramatic flooding. Although these periods were short, the effects were severe and thousands of people lost their livelihood, their restoration is estimated to take several years, and it will cost communities billions of euros.
In a few weeks, the next election on a state level will occur, and climate politics in general is a key topic in the political discussion. Good concepts for a sustainable, environmentally friendly economy can be a key point to attract new voters, since a safe livelihood and a sustainable future can be regarded as fundamental human rights. Furthermore, the required capital expenditures for a more carbon neutral economy are likely to attract investors.
The biggest challenge is to move toward full carbon neutrality in a socially acceptable way. In recent decades, the global economy has already become greener and now has a reduced carbon output. This was rendered possible by a shift in the raw material base away from coal and oil toward gas and renewables as energy and material sources as well as by increased process efficiency. Overall, this has helped to maintain or improve our lifestyle without generating more emissions. However, what we are doing is far more challenging: maintaining an acceptable lifestyle at not only constant but also highly reduced emissions.
The intergovernmental Panel on Climate Change (IPCC) stated in its most recent report: “Anthropogenic greenhouse gas emissions have increased since the pre-industrial era, driven largely by economic and population growth, and are now higher than ever. This has led to atmospheric concentrations of carbon dioxide, methane, and nitrous oxide that are unprecedented in at least the last 800,000 years.” How can the chemical industry contribute and reduce its emissions?
The natural carbon cycle that has sustained our life for thousands of years is out of control and increasing uncontrollably. The rate at which carbonaceous raw materials are transformed to finally end up as CO2 or as waste is much higher than the rate at which raw materials are regenerated.
There are several approaches to bring back some normality:
- Avoid the generation or at least the emission of CO2 wherever possible.
- Capture the CO2 that is already present in the atmosphere and transform it into persistent products that do not interfere with our global climate regulation mechanisms.
- Use CO2 as a feedstock in production processes. The carbon footprint generated from this approach will depend on the nature of the products that are made from the CO2 and on the source of energy driving the process. If persistent products are made using renewable energy sources, a negative carbon footprint is the result, which is, of course, extremely desirable.
Which of the aforementioned approaches is the most promising, and how can hte help to achieve them?
At hte, we are investigating catalytic processes in all their facets. In the short term, the optimization of existing catalytic processes is a promising route. Although the optimization of a catalyst can be achieved in a large variety of ways, at the end the optimization process it will most certainly result in a reduced carbon footprint. Roughly speaking, more active catalysts will be able to achieve the same output rate of the target product with a reduced energy input, and more selective catalysts will reduce energy intensive workup steps to obtain the target product in the desired purity.
For example, the synthesis of ammonia is one the single biggest CO2 emitters. The challenge is that the current routes to generate the required hydrogen all start from water. The water reacts with carbonaceous components and then the oxygen in the water finally ends up as CO2. Additional CO2 is produced during the combustion of carbonaceous fuels to generate the required heat input.
Ammonia is essential to sustain life, since it is the key component in fertilizers and thereby fundamental to our food supply. Ammonia is produced from H2/N2 mixtures at high pressure (>90 bar) and temperatures
(>400°C). Among all the chemicals, ammonia has the largest production capacity (200 MMT/y). Therefore, making ammonia synthesis greener would have a significant impact on the overall reduction of CO2 emissions. This can be achieved via three different routes:
- Use green H2 as feedstock, i.e. H2 that is generated by water electrolysis using electrical power from renewable sources.
- Implement a reactor heating concept that is based on green electricity instead of combustion.
- Use a catalyst that is sufficiently active at lower temperatures. Since ammonia synthesis is an exothermic reaction, the maximum possible conversion will increase with reduced temperature.
hte can certainly help in catalyst research programs aiming to improve ammonia synthesis catalysts. However, although ammonia synthesis is the biggest CO2 contributor among the base chemical production processes, by far the highest CO2 emissions are the result of the simple combustion of fossil based raw materials, either in the transport or energy sector. A substantial decrease in net CO2 emissions can only be achieved if green alternatives for these two sectors are developed.
What are some green alternatives in the transport and energy sectors that you can share about with us?
The first topic that I would like to discuss in this context is synthetic fuels. There are two major routes yielding the production of synthetic fuels. The first one is the conversion of methanol to a mixture of hydrocarbons. The reaction was discovered in 1970 by researchers at Mobil, which was at the time of the first oil crisis, during which the fuel prices increased significantly within a relatively short period of time. Methanol production from various non-oil sources was already established at that time. The process was commercialized in 1990 in New Zealand but never reached a globally relevant production level. The reaction is mechanistically extremely complex and generally yields a complex product mixture. However, using the right catalysts and the right process conditions leads to the formation of a mixture with fuel-like properties.
The second route is the Fischer-Tropsch (FT) route. This reaction was discovered in the 1910s, and quickly commercialized during WW2 to provide a source of fuels. With the notable exception of South Africa, this technology was not further commercially developed for decades, since FT based fuels were never in the position to be competitive with those based on oil.
Since the mid-1990s, there is a renewed interest in synthetic fuel production by the FT route, and several production sites have been deployed during the past decade. They mainly use natural gas as the starting material in the value chains to produce synthesis gas. Although the production of fuels by the FT route starting from natural gas is certainly cleaner than production from oil, if the process still uses fossil raw materials, it cannot be regarded as CO2 neutral.
The situation changes dramatically when the MeOH synthesis and the FT synthesis use CO2 as the starting material. Methanol synthesis from CO2 is a known process and can be run using standard, Cu based MeOH synthesis catalysts, albeit with lower activity compared to the commercial MeOH synthesis process using CO and CO2 mixtures. FT synthesis from pure CO2 has been occasionally reported in the literature, but generally suffers from high methane selectivity. However, CO2 can be converted to CO by selective reduction with H2, thereby producing water as a byproduct. The CO can subsequently be used as feedstock in a conventional syngas conversion process.
Although the reaction of CO2 and H2 to CO and H2O, as a reverse water gas shift reaction has been known for a long time, it has not received nearly as much attention as the backward reaction, namely the water gas shift. Finding selective and active catalysts for the conversion of CO2 to CO is certainly a powerful enabling technology toward CO2 neutral fuel production.
Regardless of whether the MeOH route or the rWGS/FT route is used to produce hydrocarbons, the use of CO2 instead of CO requires one additional mol H2 per mol converted CO2. The availability of green hydrogen is, therefore, a fundamental requirement for the sustainable production of green fuels.
The second topic is electrification. Currently, battery powered vehicles are very actively promoted in the public discussion to achieve CO2 neutral mobility. Although EVs can indeed be operated with zero emission during their use, their overall CO2 footprint depends on a number of factors, most importantly the source of the electric power used for charging their batteries, and the environmental footprint associated with the mining of the raw materials use for battery production, such as cobalt and lithium.
One clear benefit of synfuels based mobility compared to electric mobility is that synfuels can be used for the existing fleet, without the need for a new generation of vehicles. Furthermore, synfuels have a far higher volumetric energy density and much higher charging rates than batteries, which is crucial for aviation, for example. Nevertheless, with the broad availability of green electricity and sustainable manufacture and recycling of batteries with high capacity and high charging rates, the dream of green mobility can become a reality. However, further research and development in an interdisciplinary fashion is needed to reach that goal.
Considering the time scale, a change from a fossil to renewable raw material basis will not happen in a disruptive manner. What transition technologies can enable the switch?
Indeed, the path of the global economy from its current state to a fully CO2 neutral state would require a large number of small steps, with the contribution of all of us. As mentioned hereinabove, this transition is already in progress with a shift in the raw materials base from coal over oil to gas.
In general, the more successful a transition technology is, the better it combines the requirements of CO2 neutrality, on the one hand, and compatibility with the existing assets, on the other hand. Synthetic fuels that would keep the existing fleet in operation is a good example.
One general approach to reduce CO2 net emissions is carbon capture (CC) technologies, which consist of the selective removal of CO2 from flue gas streams before they are emitted into the atmosphere, or from ambient air. There is no “one size fits all” CC technology, since the CO2 comes in a broad range of process conditions (pressure and temperature) and accompanied by a large variety of matrix components (oxygen, moisture, inert gases, hydrogen, organic components from non-polar to polar).
Considering the importance played by H2 in the reduction of carbon emissions, making the H2 production greener is a powerful transition technology. As aforementioned, the dominating technology for the production of H2 is the reforming of carbonaceous raw materials, inevitably producing CO2 along the way. The H2 produced thereby is called “gray hydrogen”. If gray hydrogen is used for CO2 conversion, either by methanol synthesis or rWGS, the impact on the CO2 footprint will be neglectable.
However, if the reforming step uses biomass or natural gas, and if the produced CO2 is captured, the produced “blue H2” can be used to “neutralize” CO2. Any development on the catalysts or the process side that makes the production of blue H2 more energy or material efficient will be a powerful bridge technology, until “green H2”, which is produced by water electrolysis using renewable electricity, is available on a large scale.
However, one requirement for CC is that suitable materials exist with the ability to selectively adsorb CO2. Ideally, the adsorber material should be tailored to allow the later release or desorption of CO2 to make it accessible for chemical conversion reactions. At present, liquid organic amines are the most frequently used CO2 capture agents. However, the release of CO2 from these materials is an energy consuming process that reduces the positive impact of the capture process on the CO2 footprint. Solid CO2 adsorber materials are regarded as superior to liquid ones, due to their easier handling, extended thermal operating range, and limited leakage into the environment.
Developing a more effective CO2 adsorber with the easier recovery of the adsorbed CO2 could lead to a further bridge technology. Characterizing materials by their CO2 adsorption and desorption behavior requires a different testing approach than traditional screening and the testing of heterogeneous catalysts. In standard testing, the response of catalysts to changes in the process conditions or changes over time is monitored. Investigating adsorber materials, however, requires identifying the point when a material has been fully saturated, changing the process conditions to release the adsorbed material, and following the desorption process.
Interestingly, hte has developed suitable workflows for this type of processes, including experimental workflows with repeated changes in process conditions, analytical methods to track the concentration of the sorbate during adsorption and desorption, and evaluation concepts to assess storage capacities and cycle stabilities. We can, therefore, support research in optimized CO2 capture materials.
The EU plans to encourage companies to recycle their products. Will the chemical industry participate in a circular economy approach?
Most chemical companies have already committed to becoming more “sustainable” in one way or another. CO2 neutrality is the most actively promoted goal, but sustainability also entails efficient recycling strategies and processes.
As demonstrated hereinabove, by far the biggest source of overall CO2 emission is the release of stored chemical energy through combustion. For example, mobility is, from a chemical point of view, the combustion of hydrocarbons to carbon dioxide and water in order to subsequently use the released energy in the form of mechanical work. In the same way, our daily life and consumption are simply the conversion of value-added products to waste.
Production processes involving recycled materials are generally energy intensive and can easily have a bigger carbon footprint than processes starting from oil and gas when all the factors of the production process are taken into account. For example, recycling primarily means the collection of widely distributed small batches of material, and the resources allocated to the collection process add up to the carbon footprint. With sustainable methods of energy production and mobility, this disadvantage could be alleviated or even eliminated. Then, the fundamental benefit of recycling processes, namely the far better conservation of resources, will fully pay off.
Activities in the industry for more material efficient production processes are thus complementary to activities aiming to reduce the CO2 footprint. New, more sustainable products resulting from these activities generally have a high market acceptance despite their premium price. I am, therefore, convinced that the commitment of the industry to the circular economy will increase in the foreseeable future.
The biggest challenge of the circular economy is the feedstocks. Waste feedstocks are frequently mixtures with several phases that tend to separate and contain corrosive components, such as halogens, or unconventional components, such as metals or other inorganic residues.
At hte, we are frequently confronted with unconventional feeds that are oftentimes challenging to process. Due to their variable composition and molecular complexity, they may also contain significant amounts of catalyst poisons that require the development of new types of catalysts for the upgrading process. However, the awareness of these issues will increase over time and the ease of recycling will play an increasingly important role in the design of new materials.