Solutions for HVO Renewable fuels: From pre-treatment to hydrotreating
By: Bent Sarup
With an ever-increasing focus to reduce CO2 emission and closing in on the gap to become carbon neutral, the interest and pressure to produce biofuels is higher than ever. Most biodiesel today is produced as FAME (Fatty Acids Methyl Esters) through the liquid phase transesterification reaction between acylglycerides (main components of vegetable and animal fats and oils) and methanol under mild conditions. FAME as blend component to petroleum diesel has served industry and society well for many years, but has significant limitations with respect to blend ratio. Another significant drawback of the transesterification route is that jet fuel cannot be produced with that technology.
Instead, green diesel or jet fuel produced through hydroprocessing / isomerisation of fossil-free feedstock, or so-called HVO processing (Hydrotreated Vegetable Oil) has emerged as a more viable solution. With HVO processing, production capacities of more than 20,000 bbl/day are achievable, if required in parallel pre-treatment trains (projects up to 60,000 bbl/day announced), with both green diesel and jet-fuel produced with the same or even better qualities than traditional petroleum-based fuels. Pre-treatment systems can be designed for a wide range of feedstocks ranging from vegetable and animal fats and oils, via waste oils & fats to co-processing of second generation feedstocks from pyrolysis or hydro-pyrolysis oils generated from bio mass.
Due to these advantages, many refinery operators are today implementing HVO processing in their existing refineries, through drop-in co-processing of bio-based feed stock in existing Diesel Hydrotreating (DHT) units, or by revamping an existing DHT to become a dedicated HVO processing unit or by integrating a new grass-root HVO processing unit in the plant. On top of this, entrepreneurial companies are entering the fuels market constructing their own stand-alone HVO complexes.
Investments in an HVO complex is typically a magnitude higher than for a traditional FAME plant and will commonly be designed for a high capacity to get an advantageous economics of size. For a complete stand-alone HVO complex following process units are required:
- Pre-treatment unit (PTU) of bio-based feedstock,
- Hydrogen production unit (HPU),
- the HVO process itself,
- Sulphur Recovery Unit (SRU) including Amine Treatment Unit (ATU), Tail Gas Treatment Unit (TGTU) and Sour Water Stripper (SWS) and
- Waste Water Treatment Unit
With the exception of the PTU unit, these processes are typical “refinery processes”, but the feedstock is different and that poses new challenges to the refinery operators to maximise plant profitability by achieving maximal feedstock flexibility, maximising plant cycle length and product yield and flexibility, minimising risk of equipment corrosion and fouling as well as optimising utility consumption (energy and water) and waste handling.
In the sections below, some of these challenges will be highlighted, together with solutions and considerations necessary to maximise profitability from HVO processing complexes.
The pre-treatment unit
One of the most important success factors of the HVO complex is for sure the Feed Pre-treatment unit. Impurities vary significantly between feedstocks as shown in Table 1.
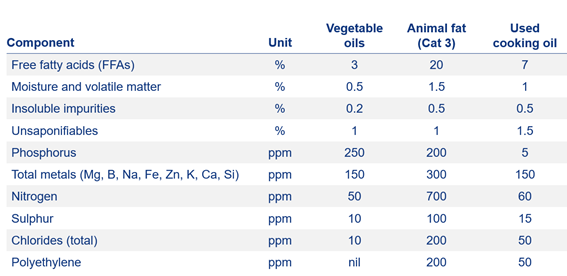
Without proper feed pre-treatment, impurities present in bio-based feedstocks will create issues such as equipment fouling and corrosion and reduced catalyst cycle length and selectivity, as seen in Table 2.
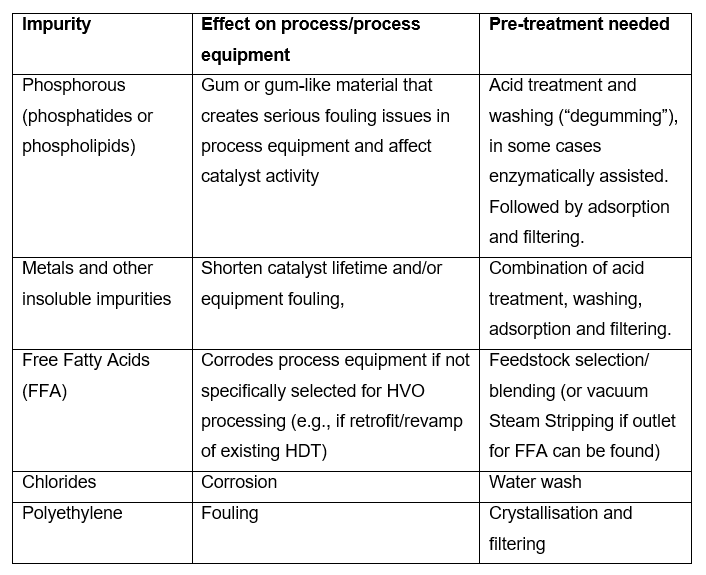
Depending on what feed-stock is going to be used in the HVO process, and if the HVO plant is a revamped old DHT unit or an on-purpose new-built process unit, different pre-treatment processes are needed. For maximal flexibility in feedstock selection, the investment in the pre-treatment unit must be tailored to meet these varying needs. Other parameters deciding what feed pre-treatment processes selected are environmental legislations, value and handling cost of by-products and utility and labour cost and availability.
Phosphorous removal is key and process design depends on the type and contents of phospholipids in the feedstock (Table 3).
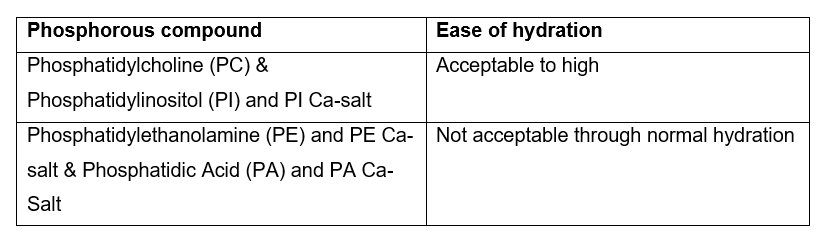
Typically, the oil is treated with an acid (such as phosphoric or citric) for a high conversion of oil soluble phospholipids (the “non-hydratable” phospholipids) to their water soluble form, which can then be separated efficiently in the heavy phase in high speed separators. For deep removal of non-hydratable phospholipids enzymatic de-gumming can be applied to convert the phospholipids to lyso-phospholipids (cutting off a fatty acid side chain) and increase their water solubility. Enzymatic degumming is already widely applied for pre-treatment upstream FAME biodiesel production units and now also seen implemented in several HVO pre-treatment designs.
The degumming process produces a significant amount of waste water, typically 5 – 10% of the oil flow, which needs to be treated as described in below section.
Acceptable phosphorous content in the feedstock before sending it to the HVO process is typically less than 3 ppm and to reach such value, along with low levels of other impurities such as metals, an additional adsorption step is required. After this process section the final pretreated oil quality is achieved (Table 4).
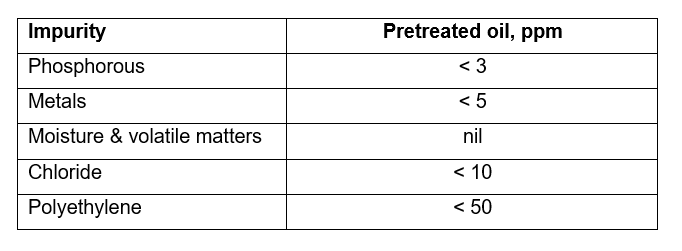
In brief, in the adsorption section the oil is contacted with typically 0.5 – 2.0% adsorbent material (“bleaching clay”) that after a proper contact in a stirred slurry adsorption vessel is filtered from the oil in pressure leaf filters. Various qualities of bleaching earth/clay exist in the market and price and performance is normally related. This process is fully automated and continuous.
In some cases, as per Table 1 above, additional process sections upstream the degumming and adsorption sections are required to bring down chloride (by washing) and polyethylene (by crystallisation/filtering) contents to the pretreated oil purity shown in Table 4.
In some cases, the FFA may need to be adjusted due to potential downstream corrosion issues. Although in principle possible to select metallurgy for 100% FFA feed, most existing HDT units will be required an upper acceptable FFA content, such as 10 or 20%. This is most economically achieved by feedstock selection and blending, but the option exists to implement a de-acidification process using steam stripping under vacuum in cases with an outlet for the FFA and ample access to low priced, high FFA feedstock.
In summary the pre-treatment processing options can be displayed as shown in Figure 1.
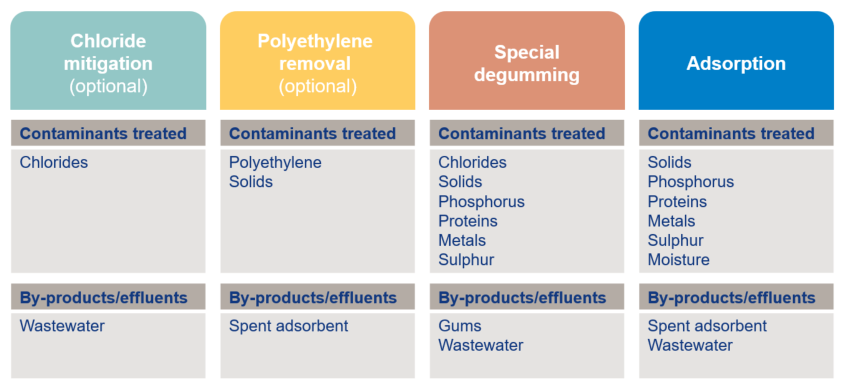
Effluents and treatment
In summary, the main effluents from the pre-treatment unit will be used bleaching clay and waste water mainly from oil washing operations. As always handling of effluents will depend on local site conditions. Such as spare capacity in an existing waste water facility, specifications for discharged, cleaned waste water etc.
Spend bleaching clay will hold in the order of 20 – 25% residual oil. Examples of disposals could be for biogas manufacture, companies extracting the residual oil, or burning the clay for the fuel value in the residual oil.
Upgrading waste water facilities could include debottlenecking existing facilities or add new treatment facilities using well known technologies such as dissolved air flotation (DAF), sequencing batch reactors (SBR) as activated sludge process, aerobic digesters, decanters, high-speed centrifuges. Or implementing a Zero Liquid Discharge technology using multiple effect evaporators and recycle evaporated water for process usage.
Conclusion
HVO is definitely making its entry in the petroleum refineries and many facilities are already on-stream. The future will hold a quest to maximise usage of waste and non-edible oil sources and the pre-treatment facilities designed to maximise feedstock flexibility to handle as much as possible of such feedstocks.

About the author
Dr. Bent Sarup holds a PhD and a Master’s degree in Chemical Engineering, as well as an executive MBA degree. He has authored or co-authored more than 30 technical articles, a frequent presenter at conferences and inventor or co-inventor of several patents.
Dr. Sarup has 34 years of industrial experience in applied R&D, scale-up work, project engineering and management as well as sales and marketing. The industries he worked in include petrochemical and petroleum refining industries, within the last 15 years also in the edible oil refining and biofuel industries. He has a deep interest in business and organizational development coupled with technology development, and development work involving multiple organizations such as universities, customers and suppliers.